Introduction
In the pharmaceutical industry, the selection of appropriate materials for components used in critical environments plays a significant role in ensuring the performance, safety, and longevity of equipment. AISI 316LVM (Vacuum Melted Stainless Steel) and titanium alloys are two widely recognized materials for pharmaceutical applications due to their superior corrosion resistance, biocompatibility, and mechanical properties. However, each material comes with its own advantages and limitations. This article provides a detailed comparison between AISI 316LVM and titanium alloys, focusing on their applications, tolerances, surface treatments, and reasons for their respective use in pharmaceutical environments, based on standards such as ASME BPE 2024 [1], ASTM F138 [2], and ISO 10993 [3].
Do you want more info on our CNC services? Click here: CNC Engmotion Services
Do you want to ask for CNC Services? Click here: CNC Services Form
Do you want more info on our CNC services? Click here: CNC Engmotion Services
Do you want to ask for CNC Services? Click here: CNC Services Form
1. AISI 316LVM Overview
1.1 Material Composition and Properties
AISI 316LVM is a vacuum melted version of AISI 316L stainless steel, ensuring higher purity and improved corrosion resistance. The low carbon content (<0.03%) minimizes carbide precipitation, reducing the risk of intergranular corrosion during repeated sterilization cycles [1]. The molybdenum content (2-3%) enhances its pitting and crevice corrosion resistance, making it suitable for environments with chloride-rich solutions, commonly encountered in pharmaceutical manufacturing [4]. With a tensile strength of 485 MPa, AISI 316LVM strikes a balance between strength and corrosion resistance, allowing it to perform well in most sterile applications [5].
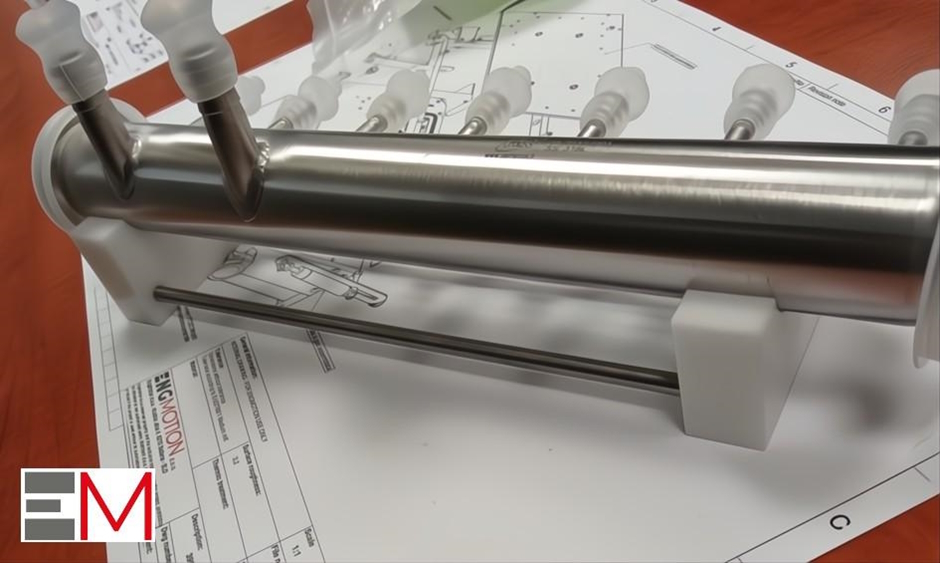
1.2 Tolerances and Machinability
In high-precision pharmaceutical components, such as volumetric pumps without seals, tight tolerances between 0.001-0.004 mm are essential to avoid leakage and ensure fluid handling precision [6]. AISI 316LVM can achieve these tolerances through CNC machining, but requires optimized cutting parameters (cutting speeds of 150-300 m/min) and carbide tooling to manage its work-hardening properties [7]. For less demanding applications, such as manifolds or piping systems, tolerances of 0.1 mm are acceptable, allowing for faster production times [8].
2. Titanium Alloys Overview
2.1 Grades and Mechanical Properties
Titanium alloys, especially Grade 2 (commercially pure) and Grade 5 (Ti-6Al-4V), are known for their outstanding biocompatibility and corrosion resistance, even in highly aggressive environments [9]. Titanium naturally forms a dense, stable oxide layer that resists corrosion in saline and acidic environments, making it highly desirable in systems exposed to chloride-rich or sterilization solutions [10]. The tensile strength of titanium Grade 5 reaches up to 895 MPa, far exceeding that of AISI 316LVM, which makes it suitable for lightweight, high-strength components in dynamic pharmaceutical systems [10].
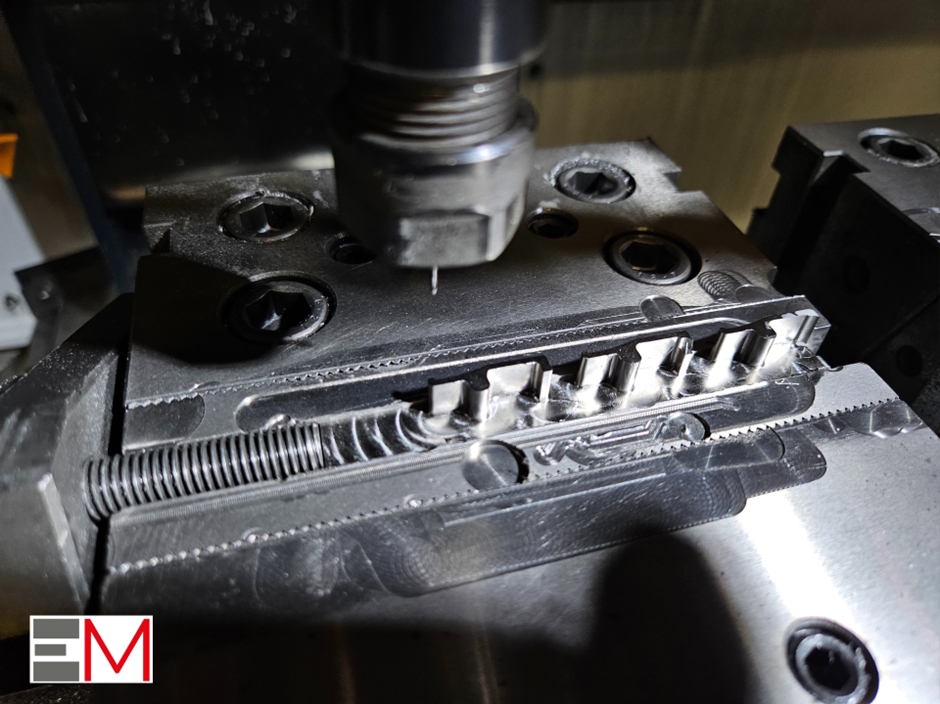
Figure 2 Special Titanium Component CNC machining
2.2 Tolerances and Machining Challenges
While titanium alloys offer excellent mechanical properties, they are notoriously difficult to machine due to their low thermal conductivity, which can lead to excessive tool wear and surface damage [11]. Achieving the same tight tolerances required for high-precision components (e.g., 0.001-0.004 mm) in titanium requires slower cutting speeds (20-60 m/min) and the use of specialized cutting fluids [12]. This not only increases production times but also raises costs due to the frequent need for tool changes and higher energy consumption [12]. For simpler components, such as static connections or supports, standard tolerances of 0.1 mm are more easily achieved, though machining remains challenging compared to AISI 316LVM.
3. The Role of Titanium in Pharma: Why Isn’t It Widely Used?
Despite its highly desirable properties, titanium has limited use in the pharmaceutical industry for several reasons:
- Cost: Titanium is significantly more expensive than AISI 316L stainless steel, not only in terms of raw material costs but also due to the complexity of machining it. The need for specialized tooling, slower cutting speeds, and frequent tool changes results in increased production costs [12].
- Machinability Issues: As mentioned, titanium’s low thermal conductivity and its tendency to gall require slower, more precise machining processes, which increase manufacturing times. This adds to the cost and complexity, particularly in high-precision Pharma components where tolerances are critical [11].
- Specific Use Cases: Titanium is most beneficial in highly corrosive environments, such as systems exposed to saline, hydrochloric acid, or chlorinated cleaning agents. However, for many pharmaceutical applications, AISI 316LVM offers sufficient corrosion resistance at a lower cost, making it the more economically viable choice [13].
- Regulatory Complexity: While titanium’s biocompatibility is well-recognized, the lack of extensive long-term data in certain Pharma applications compared to AISI 316LVM has led to a slower adoption rate. AISI 316LVM, being more established, often meets the required regulatory approvals more easily [14].
4. Comparative Analysis: AISI 316LVM vs Titanium Alloys
4.1 Corrosion Resistance
Both AISI 316LVM and titanium alloys offer excellent corrosion resistance. AISI 316LVM is well-suited for most pharmaceutical environments, especially those involving low to moderate chloride concentrations. However, in environments with highly aggressive saline or hydrochloric acid solutions, titanium’s naturally forming oxide layer provides far superior protection [13]. Titanium can withstand prolonged exposure to such environments without suffering from pitting or crevice corrosion, where AISI 316LVM might eventually degrade [14].
4.2 Biocompatibility
Both AISI 316LVM and titanium alloys are recognized for their biocompatibility, with both materials being commonly used for medical devices and implants. However, titanium offers an edge in long-term biocompatibility for applications that involve direct and prolonged contact with tissue or fluids. This makes titanium a preferred material for implants or components that require extended exposure to biological environments [15]. In contrast, AISI 316LVM is often chosen for less invasive pharmaceutical equipment, such as non-contact surfaces or external components [16].
4.3 Cost and Availability
AISI 316LVM is more readily available and cost-effective than titanium alloys, making it the preferred choice for large-scale pharmaceutical manufacturing. For applications where weight savings or superior corrosion resistance are not critical, AISI 316LVM provides sufficient performance at a lower cost [17]. Titanium, on the other hand, justifies its higher cost in applications where superior corrosion resistance, strength, or biocompatibility is required, particularly in high-performance, dynamic pharmaceutical systems [18].
5. Surface Treatments for AISI 316LVM and Titanium Alloys
5.1 Electropolishing and Passivation for AISI 316LVM
AISI 316LVM benefits from standard surface treatments, including electropolishing and passivation, to further enhance its corrosion resistance and reduce surface roughness. Electropolishing removes material from the surface to smooth out microscopic imperfections, achieving roughness values as low as Ra ≤ 0.4 µm [19]. Post-electropolishing passivation is typically performed using nitric or citric acid to enhance the natural chromium oxide layer, following ASTM A967 guidelines [12].
5.2 Anodization and Coating for Titanium Alloys
Titanium alloys are typically treated through anodization, which thickens the oxide layer on the surface, increasing corrosion resistance and wear characteristics. The anodization process involves applying a voltage (typically between 20-100V) in an electrolytic bath, resulting in a highly protective surface layer [20]. In dynamic systems that require friction reduction, titanium may also be coated with low-friction materials such as PTFE or PVD coatings, which enhance the wear resistance of moving parts [21].
Conclusion
AISI 316LVM and titanium alloys both offer excellent properties for pharmaceutical applications. AISI 316LVM is highly suitable for general-purpose components, providing a balance of cost, machinability, and corrosion resistance. Titanium alloys, while more expensive, excel in high-performance environments requiring superior corrosion resistance, biocompatibility, and strength. Material selection must be guided by the specific application, balancing technical requirements, cost, and long-term performance, all while ensuring compliance with industry standards.
Bibliography
- ASME BPE 2024. Bioprocessing Equipment Standard, 2024.
- ASTM F138. Standard Specification for Stainless Steel Bar and Wire for Surgical Implants (UNS S31673), 2013.
- ISO 10993-1. Biological evaluation of medical devices – Part 1: Evaluation and testing within a risk management process, 2018.
- ASTM A276. Standard Specification for Stainless Steel Bars and Shapes, 2021.
- S. Kalpakjian and S. Schmid. Manufacturing Processes for Engineering Materials, 6th Edition, Pearson, 2017.
- FDA. Pharmaceutical GMPs for the 21st Century – A Risk-Based Approach, 2004.
- B. Crook. The Microbiological Quality of Pharmaceuticals: Sterility, Bioburden and Data Integrity, CRC Press, 2019.
- W. Revie and H. H. Uhlig. Corrosion and Corrosion Control, 4th Edition, Wiley, 2008.
- J. R. Davis. Surface Engineering for Corrosion and Wear Resistance, ASM International, 2001.
- ASTM B348. Standard Specification for Titanium and Titanium Alloy Bars and Billets, 2013.
- M. Ohring. Materials Science of Thin Films, 2nd Edition, Academic Press, 2002.
- ASTM A967. Standard Specification for Chemical Passivation Treatments for Stainless Steel Parts, 2017.
- G. Williams et al. Corrosion Resistance of Biomedical Titanium Alloys, Journal of Biomedical Materials Research, 2018.
- A. Kamachi Mudali et al. Corrosion of Titanium Based Alloys in Biomedical Applications, Corrosion Reviews, 2010.
- ASTM F136. Standard Specification for Wrought Titanium-6Aluminum-4Vanadium ELI (Extra Low Interstitial) Alloy for Surgical Implant Applications, 2013.
- ASTM F67. Standard Specification for Unalloyed Titanium for Surgical Implant Applications, 2011.
- B. Ravi Kumar et al. Comparative Study on Titanium and Stainless Steel in Biomedical Applications, Journal of Advanced Materials and Processes, 2019.
- C. Leyens and M. Peters. Titanium and Titanium Alloys: Fundamentals and Applications, Wiley-VCH, 2003.
- R. K. Gupta et al. Surface Finish and Contamination Control in Bioprocess Equipment, BioPharm International, 2020.
- M. Ohring. The Materials Science of Thin Films, 2nd Edition, Academic Press, 2002.
- T. S. Sudarshan. Surface Modification Technologies XV, Technomic Publishing, 2002.
- B. Crook. Sterility and Surface Engineering in the Pharmaceutical Industry, CRC Press, 2019.
- Pharmaceutical Industry Case Study: Titanium and Stainless Steel in High-Precision Pharma Filling Systems, 2022.